‘There is increasing Evidence That Endocrine Disruption (EDC) Exposures Play a Substantive Role In Disease Causation Or Progression.’ Gore et al 2015
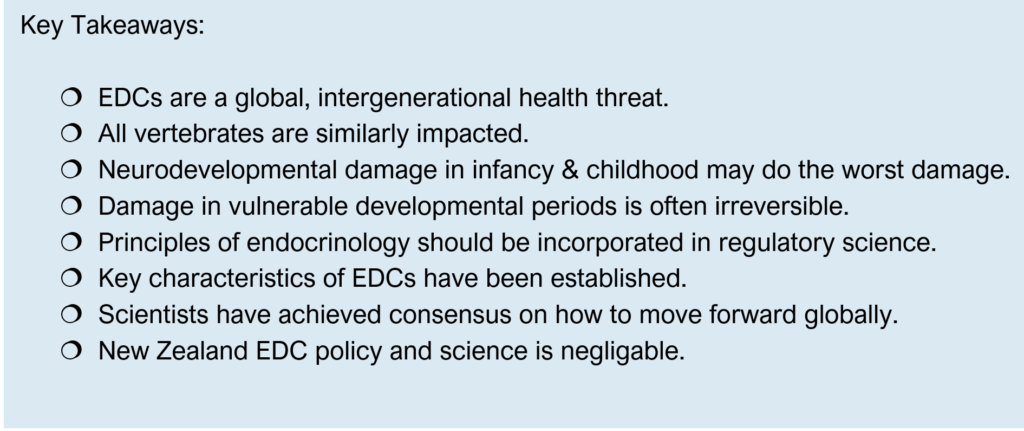
An enormous quantity of medical literature involves the manipulation of synthetic chemicals at ultra-low doses – parts per billion, for example – to alter hormone function. Hormonally active drugs are actively developed and marketed, specifically designed to effect hormone function at parts per billion and parts per trillion.
Non-voluntary exposures – from diets, air, workplaces, household, personal care products and other commercial products – to endocrine disrupting compounds at environmentally relevant levels can alter gene-environment interactions via physiological, cellular, molecular, and epigenetic changes. These chemicals are hormone disruptors, or endocrine disrupting compounds (EDCs):‘an exogenous chemical, or mixture of chemicals, that interferes with any aspect of hormone action’ (Gore, et al., 2015).
Yet the science on EDCs lags exponentially behind the science on hormonally-relevant medicine. When governments and regulatory agencies do not keep up to date with complex issues, based on the best available science, their actions have the effect of framing out ignorance and uncertainty. It begs the question – is the ‘proof’ required to pull an EDC from the market considerably more onerous than the ‘proof’ required to get a drug to market?
Technocracy is art of separating out interlinked scientifically recognised issues, and ignoring inconvenient data through dismissal or simply pretending it doesn’t exist. Technocratic activities always acts to delay or prevent regulation of a human or environmental health harm in the public interest. Regulatory agencies have been slow to adopt EDC principles and to compulsorily require independent data on EDCs. It is the potential for additive and synergistic effects to produce ongoing harm ‘cascades’ that both accumulate over a life-time and over generations that is of greatest concern. Scientists contest that EDC effects can be ‘separated out’ – that scientists can delineate EDC harms from other life exposures (Demeneix & Slama, 2019; Kassotis, et al., 2020).
However, without building methods to assist regulators and scientists to navigate uncertainty (which is a function of open systems) regulators find themselves in a double bind, where they can never know the precise ‘quantitative’ level of risk – so they are slow to act and therefore delay regulation (Honkela, Toikka, Hukkinen, & Honkela, 2014).
EDCs have emerged as a global health threat.
An established body of evidence demonstrates that daily exposures to predominantly manmade (anthropogenic) chemicals disrupt the endocrine system, by mimicking, perturbing and/or blocking hormone function, and harming health.
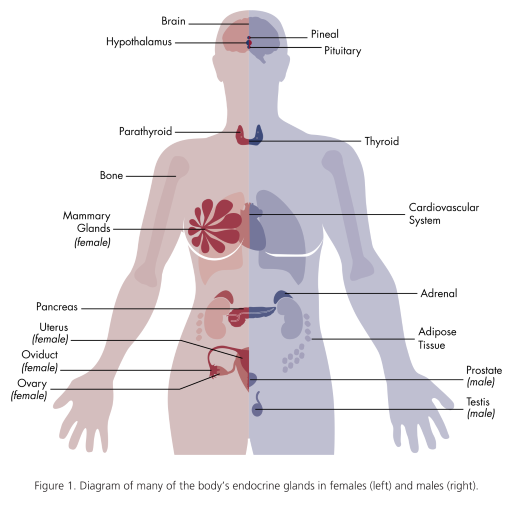
EDCs do the most damage before conception and during developmental stages because exposures in this time can result in permanent damage, and the social and economic costs aggregate, particularly when intelligence and behaviour are affected (Demeneix, 2017). EDCs not only impact our chances of conceiving (Green, Harvey, Finger, & Tarulli, 2021), environmental chemicals cross the placental barrier and damage babies in utero (prenatally).
Babies are born ‘pre-polluted’ (NIH, 2010) and a foetus can be exposed to 300 chemicals (Bourguignon, et al., 2019). Infants and children consume more by bodyweight, and as a result, have higher levels of EDCs in their bodies. Tests in children’s urine, blood serum or hair demonstrate higher levels of anthropogenic chemicals, including EDCs (‘t Mannetje, Coakley, & Douwes, 2018; Iglesias-González, Hardy, & Appenzeller, 2020). Testing across countries demonstrates a direct relationship with regulatory restrictions and exposure levels (Attina, et al., 2016).
Endocrine disrupting compounds (EDCs) have been detected in air, dust, food and food packaging, water, personal care products, household goods, detergents, fabrics and upholstery, electronics, medical equipment, pesticides, air and water (Gálvez-Ontiveros, Páez, Monteagudo, & Rivas, 2020; Kassotis, et al., 2020; Metcalfe, et al., 2021). The inability of waste-water and drinking water managers to effectively strip EDCs from water (Kasonga, Coetzee, Kamika, Ngole-Jeme, & Momba, 2021) ensures that persistent EDC compounds will circulate through human and environmental food webs.
Vertebrates are similarly impacted
Endocrine disrupting compounds can harm function across vertebrate systems as hormones have the same chemical structure across species. Therefore, EDCs can alter functioning of the immune system; reproductive system (including altering fertility, fecundity and sexual characteristics); the nervous system and brain and drive hormonally relevant cancers (Colborn, Myers, & Dumanoski, 1997; Demeneix & Slama, 2019). Particularly harmful exposures can occur preconception, altering fertility and fecundity with less fertile individuals or couples having higher EDC levels (Green, Harvey, Finger, & Tarulli, 2021).
The similar activity (and risk profile) of EDCs across vertebrate systems is well-recognised in the scientific literature. While for example, in many instances carcinogens work differently across vertebrates, EDCs work remarkably similarly – as Colborn and colleagues (1997) outlined some 25 years ago:
‘As scientists have explored hormone receptors in different animals, they have marvelled at the lack of change over millions of years of evolution. Whether a turtle, or mouse, or human, the endocrine system produces a chemically identical estradiol that binds to an estrogen receptor. The discovery of similar estrogen receptors in animals as distinct as turtles and humans suggests that the internal communication system based on hormones and receptors is an ancient adaption that arose early in the evolution of vertebrates- the evolutionary branch of animals with backbones that includes humans. Scientists believe that turtles have undergone little change since they arose from a reptilian ancestor over 200 million years ago, long before modern mammals appeared on the scene.’ (Colborn, Myers, & Dumanoski, 1997, p. 74).
Perhaps the most concerning EDC risk across vertebrate species are the impacts on cognitive functioning, intelligence and behaviour (Attina, et al., 2016; Nesan & Karrasch, 2020). Exposures may be associated with increasingly common disorders, including attention-deficit hyperactivity disorder, global developmental delay, autism spectrum intellectual disability and other communication neurodevelopmental disorders (Rivollier, Krebs, & Kebir, 2019). Exposures during pregnancy are associated with neurodevelopmental delays (Tanner, et al., 2020).
While receptor binding is the most common feature of EDCs (Vandenberg, 2019), exposures that adversely alter one system can lead to cascading effects in across the body. For example, the developing immune system is tightly regulated and highly sensitive to exposures and impacts from developmental immunotoxicity, may trigger systemic risks due to the close interactive relationship of the immune system with many other body systems (DeWitt & Patisaul, 2018).
The thyroid & intelligence
‘The thyroid may be target of more chemicals than any other endocrine system’ (Demeneix, 2017, p. 7).
Professor Barbara Demeneix has drawn attention to the extreme vulnerability of thyroid function and the problem of a large spectrum of anthropogenic chemicals to alter and perturb thyroid function. The right amount of thyroid hormone, at the right time is critical for brain development and function. The thyroid has a central role in intelligence – and as Demeneix (2017) has noted
‘I still receive incredulous comments and questions about how an amphibian model, a tadpole, can be relevant to humans’… ‘the best answer to those doubts comes from the reputed French thyroid clinician and researcher Jacques Legrand, who is reported to have pointedly said “Without a minimum of thyroid hormone at the right time, a tadpole fails to become a frog and a human baby becomes a cretin.” Yes, exactly the same molecule that is needed to orchestrate the metamorphosis of a tadpole into a frog is required for optimal brain development in all vertebrates, including humans.’ (Demeneix, 2017, p. 30)
Migration of neurons into brain architecture; myelination and synapse formation all require the optimum amount of thyroid hormone to occur. Thyroid levels in pregnancy are intimately related to offspring intelligence, behaviour and motor function. The critical sensitivity of these processes has been emphasised by Barbara Demeneix ‘[t]he effects of thyroid hormone deficiency on brain development is so marked that hypothyroidism in gestating rats can be used to study autism-like symptoms in the pups’ (Demeneix, 2017, p. 37). Autism levels in the human population have increased, and Demeneix emphasises that only a quarter of the increase is accounted for by diagnostic change (p. 6).
The mechanisms through which EDCs interference with thyroid function are increasingly documented, though data gaps remain. EDCs interfere across the thyroid system, disrupting the central regulatory system in the hypothalamus and pituitary, thyroid hormone production at the thyroid gland, thyroid hormone transfer, as well as hormone bioavailability, function, and metabolism (Ghassabian & Trasande, 2018; Preau, Fini, Morvan-Dubois, & Demeneix, 2015). Developmental exposures may not only impact neurodevelopment but affect immune response, due to cross-talk between the endocrine and immune systems (O’Shaunghnessy, Fischer, & Zenclussen, 2021).
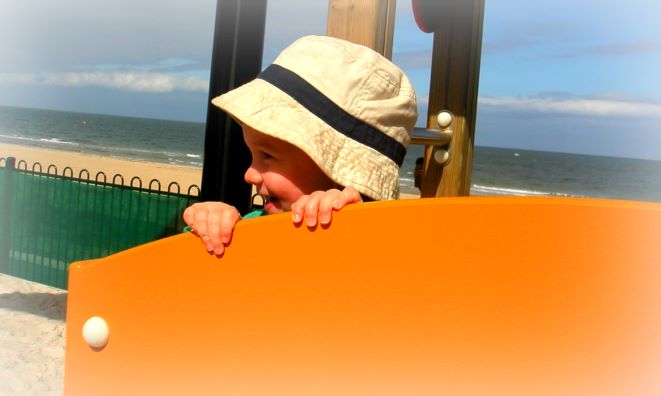
Demeneix acknowledges the interdependency of the thyroid function for neurodevelopmental function on not only absence of hormone disrupting chemicals, but on adequate nutrition. The main cause of brain damage in childhood is iodine deficiency, impairing cognitive and motor development (WHO, 2013). Yet levels of chemicals normally considered benign by toxicological standards, can impact thyroid function. Fluoride can disrupt iodine take-up and accumulation (Waugh, 2019). Toxicological analyses of fluoridation for dental purposes do not involve consultation with endocrinologists to evaluate neurodevelopmental risk, nor consider the potential for waste-water to pollute rivers and impact the hormone functioning of aquatic vertebrates (PSGRNZ, 2021).
There is compelling evidence that chemical pollutants are significantly contributing to the overwhelming and undeniable increase in hormone, or endocrine related cancers and other chronic diseases disabling world populations today, which did not occur in the generations before.
Quite simply, genes don’t change that quickly, they are only directly responsible for some 10-20% of illness.
Approximately ninety percent of illness is driven by environmental stressors (Rappaport & Smith, 2010; Rappaport, 2016), yet the vast majority of health research is steered towards genetic and molecular research for drug development (Vermeulen, Schymanski, Barabási, & Miller, 2020).
Metabolic Disorders
Data on EDC is increasingly challenging the narrative that genetics, diet, exercise and aging are the dominant drivers of the current epidemic of metabolic disease (Heindel, et al., 2017; Kumar, et al., 2020; Papalou, Kandaraki, Papadakis, & Diamanti-Kandarakis, 2019).
As EDCs are implicated in the development of metabolic disorders – scientists have identified an EDC subset class, the metabolism disruptors (Heindel, et al., 2017). Metabolic syndrome, represented by a complex set of conditions including insulin resistance, abdominal obesity, dyslipidemia, hypertension, and hyperglycemia. These conditions increase risk for cancer, cardiovascular disease, type 2 diabetes, stroke and chronic kidney disease. The tissues and hormones which control metabolism, the neuroendocrine system, adipose tissue, the organs which regulate glucose homeostasis (pancreas, liver and skeletal muscle), the thyroid, the sex/reproductive hormones can all be impacted by EDC exposures. Critically, when these pathways are disrupted during vulnerable developmental windows, the negative effects can be long-lasting and impact lifetime health and wellbeing.
Scientist argue that EDCs are an unacknowledged and underlying factor which have increased risk in the COVID-19 pandemic, as many of the underlying (and overlapping) health conditions that place people at risk of death or hospitalisation: diabetes, obesity, cancer, cardiovascular disease, and immune dysfunction – are exacerbated by exposure to EDCs from conception and across the life-course (Adegoke, et al., 2021; Gupta, et al., 2020).
Hand me down poisons
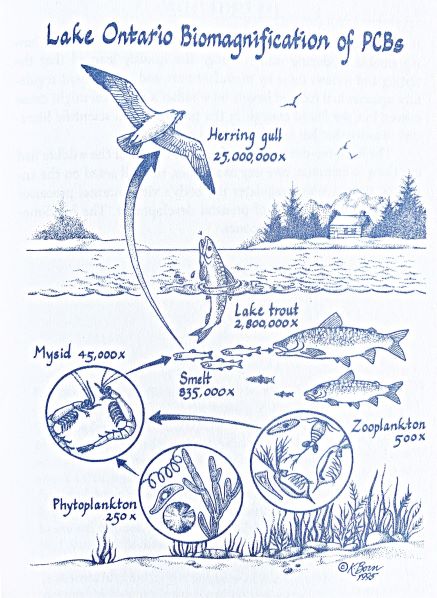
Colborn and colleagues recognised at an early stage that EDCs were ‘hand me down poisons’. The seminal 1996 Our Stolen Future book, which introduced the world to EDCs, articulated how exposures biomagnified through the food chain. First, microscopic organisms would pick up the EDCs in sediments, these would be consumed by slightly larger organisms called zooplankton, which would then be consumed by mysids (shrimp), who would be consumed by fish, and larger fish, up to the herring gull (see image below from Our Stolen Future). It was in this way that polychlorinated biphenyls (PCBs) concentrations in tissue would be found to be magnified up to 25 million times (Colborn, Myers, & Dumanoski, 1997, p. 26).
EDC effects are also ‘handed down’ through heritable changes in gene expression (nongenetic inheritance) – epigenetic alterations. These changes do not arise from alterations to the DNA sequence, rather occur epigenetically. These changes can produce intergenerational alterations in unexposed generations, which can lead to increased disease risk, or as Mark Skinner articulates, the environmentally induced epigenetic transgenerational inheritance of disease for descendants, including grandchildren (F2 generation) and great grandchildren (F3 generation) (Nilsson, Sadler-Riggleman, & Skinner, 2018; Skinner, 2016).
The irreversibility of effect during vulnerable developmental periods
Exposure to during sensitive developmental periods in utero, infancy, during adolescence and in pregnancy and menopause can increase risk for diseases. Foetuses, infants, children and adolescents have critical developmental windows, recognised as DOHaD, the developmental origins of health and diseases when ‘subtle changes in gene expression and biological molecular processes, which, ultimately, alter permanently the developmental trajectory and lead to long-lasting dysfunction’ (Papalou, Kandaraki, Papadakis, & Diamanti-Kandarakis, 2019).
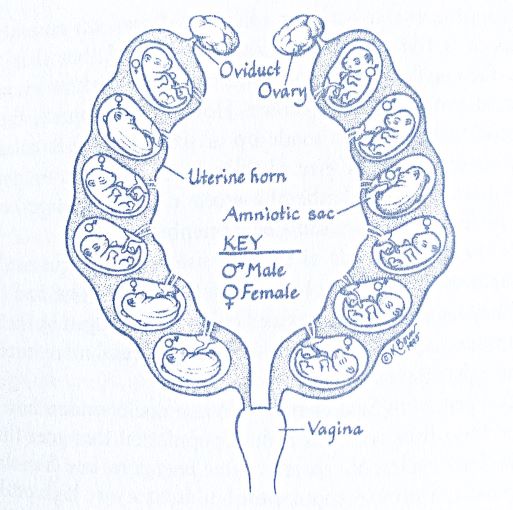
Hormone potency (the concentration of a compound that is required to produce a biological effect at a given intensity) at very low doses (part-per-billion (ppb) or part-per-trillion (ppt)) is impacted by the ligand’s binding affinity for the receptor, the number of receptors, the concentration of the hormone, and the developmental stage when exposures occur (Vandenberg, 2019, p. 13).
For example, exposure to diethylstilbestrol (DES) during pregnancy is associated with a higher risk for ADHD in the grandchildren of the exposed women (Kioumourtzoglou, Coull, O’Reilly, Ascherio, & Weisskopf, 2018).
Hormone changes have been with us a long time and exceptionally low levels of testosterone and oestrogen in utero alter sexual characteristics. An eye-opening description of the ‘wombmate effect’ illustrated by Frederick vom Saal’s (1984; 1989) work (for greater discussion and a full reference list see (Colborn, Myers, & Dumanoski, 1997)). Sisters near brothers were exposed to more testosterone in the womb, and exhibited more aggressive tendencies. Sisters near sisters produced more attractive pheromones and were more fertile than their testosterone exposed sisters. However, the sisters exposed to more testosterone would be more resilient in times of famine or overcrowding – and could carry the colony through. Male behaviour could also be altered. Oestrogen exposure before birth increased the sexual activity of male mice in adult life and these mice were more likely to attack offspring. High testosterone males with brothers for wombmates tended to parent more similarly to females. (Colborn, Myers, & Dumanoski, 1997) Rodent studies demonstrate that endogenous or exogenous exposures can impact both sexual development and disease risk in later life (Palanza, Nagel, Parmigiani, & vom Saal, 2016; Yang, et al., 2021) but also epigenetically impact sexual behaviour across generations (transgenerationally) (Kermath, Thompson, Jefferson, Ward, & Gore, 2022). While it would be misleading to directly infer that the effects on mice directly translate to human behaviour, it is now clear that human females can be masculinised in utero, while human males can be feminised in utero.
The economic costs of EDC
Scientist cohorts have explored the burden of disease resulting from EDC exposures and the economic cost to society and the cost to health from environmental exposures has been estimated to exceed 10% of global domestic product (Attina, et al., 2016; Hunt, Sathyanarayana, Fowler, & Trasande, 2016; Hauser, et al., 2015; Trasande, et al., 2016).
Grandjean and Bellanger (2017) suggest that the harmful potential of EDC exposures appear to have been under-estimated in global burden of disease (GBD) calculations, noting that the potential for EDCs to drive downstream harm are not clearly elucidated via specific medical diagnoses.
Grandjean and Bellanger cite the example of increased body mass index. Obesity is an important risk factor considered when measuring the GBD, and the GBD assessments situate environmental risk factors as a separate category, yet exposure to phthalates and other EDCs drive obesity (Alonso-Magdalena & Nadal, 2020). EDCs that drive obesity potentially produce multifactorial health risks across the life course, and reduce life expectancy. Without large global studies drawing attention to these more nuanced effects and acknowledgement of the role association-wide studies can play in uncovering knowledge, it is difficult for policy-makers to accurately gauge risk for nation-states (Grandjean & Bellanger, 2017).
Median annual health costs of exposure to EDCs in the European Union has been estimated at €157bn (or USD209 billion), equivalent to 1.23% of the continent’s GDP. The estimates were drawn from EDCs with a high probability of causation. The main health conditions associated with a greater than 20% probability of being caused by EDCs, included ‘IQ loss and associated intellectual disability, autism, attention-deficit hyperactivity disorder, childhood obesity, adult obesity, adult diabetes, cryptorchidism, male infertility, and mortality associated with reduced testosterone.’ The study authors concluded:
‘endocrine disrupting chemical exposures in the EU are likely to contribute substantially to disease and dysfunction across the life course with costs in the hundreds of billions of Euros per year. These estimates represent only those endocrine disrupting chemicals with the highest probability of causation; a broader analysis would have produced greater estimates of burden of disease and cost.’ (Trasande, et al., 2016)
A related study by Attina and colleagues (2016) looking at the disease costs from EDCs, estimated that the cost to US society was greater, at USD340 billion, equivalent to over 2% of GDP. The study authors considered that the higher US economic cost was in large part due to exposure to polybrominated diphenyl ethers, while in Europe the costs resulted from exposure to organophosphate pesticides. (Attina, et al., 2016).
Moving forward to better evaluate & regulate
Regulatory decisions and policies are yet to meaningfully decrease human exposure to EDCs and current approaches to limiting exposures in humans are ‘dangerously slow and insufficient’ (Kassotis, et al., 2020). Too few chemicals in commerce have been tested, testing does not keep up with chemical innovation and manufacture, and when harm has been identified regulatory action is hesitant or non-existent (Kassotis, et al., 2020; Persson, et al., 2021).
Risk based approaches, used by the Anglo-nations ( the U.S., Australia, Canada, New Zealand) do not consider the costs of EDCs to the burden of disease, and are unable to take into account issues such as the long period between infancy and childhood exposures and the impact on health and economic activity in adulthood (Kassotis, et al., 2020). Risk-based approaches claim to evaluate the hazards of a chemical and the anticipated exposure.
New Zealand
New Zealand has no formal policy on endocrine disrupting compounds (NZEPA, 2021) and EDCs are not prioritised in regulatory decision-making (Iorns Magallanes, 2018). There is no centre for research on EDCs and EDC research does not tend to be funded. When chemicals are detected at environmentally relevant levels, there is no scientific field that can take monitoring studies and evaluate risk, to inform the public sector.
When public science is not frequently undertaken (as in New Zealand), exposure estimates may be limited or out of date; or rely on technical estimates drawn from other jurisdictions, which does little to inform the public sector on real world local exposures. Limited biomonitoring data, and the absence of local biomarker and serum/urine testing studies which can be fed back into the regulatory environment, hinders accuracy as to exposure levels and population-level risk.
In New Zealand, when chemicals are evaluated for risk, cost-benefit analyses are predominantly supplied by the industry being regulated (NZEPA, 2018), and sometimes contracted out by the regulatory agency (Comendant & Davies, 2018). The New Zealand Environmental Protection Authority (NZEPA) analyses focus on the loss to the economy, should a chemical not be approved. New Zealand’s Risk Assessment Methodology for Hazardous Substances (NZEPA, 2020) does not discuss EDCs, and studies evaluating EDC exposures are not emphasised as critical to risk assessment in New Zealand.
Paradigm shift – incorporating endocrinology principles in risk assessment
Principles of endocrinology have not been integrated into regulatory decision-making. These principles differ from conventional toxicological approaches. Effectively, endocrinologic principles introduce a paradigmic shift in regulatory risk conventions, and can assist scientists to understand how hazard and risk assessment of EDCs – in the public interest.
EDCs are best described and understood by their mechanism of action (Barouki, 2017). Scientists have argued that principles of endocrinology (rather than toxicology) must be necessarily applied by regulatory agencies if EDCs are to be accurately identified and regulated.
Five major principles underpin the study of hormone function. From (Vandenberg, Endocrine Disruptors and Other Environmental Influences on Hormone Action, 2019):
Principle 1. Hormones are responsible for coordinating the tissues of the body from conception until death, functions include regulation of embryogenesis, foetal development, birth, puberty, pregnancy, parturition & aging.
Principle 2. Hormones act via highly specific binding to receptors. Hormone function can be mediated by the concentration of a hormone, the binding affinity and the potency of a hormone (or a mimic/disruptor).
Principle 3. Hormones act at exceptionally low doses. Most hormones circulate in the blood at part-per-billion (ppb) or part-per-trillion (ppt) concentrations. Eg. estradiol, testosterone (in adult females), & thyroid hormone circulates typically in the ppt range; progesterone & testosterone (in adult males) at ppb.
Principle 4. The effects of hormones depend on life stage. Exposures in critical developmental periods induce ‘organizational’ responses, permanently altering the development of cells, tissues, and organs.
Principle 5. Hormones display nonlinear and even nonmonotonic relationships between dose and effect. If doses at a very low dose mimic hormonal action (such as at a level of parts per billion or trillion, this may have a greater biological effect than if a substance was dosed at a higher non-hormone relevant level.
Others have sought to illustrate the difference between conventional toxicological approaches and EDC integrated toxicology. In a 2017 paper, Professor Robert Barouki illustrated many of the differences:
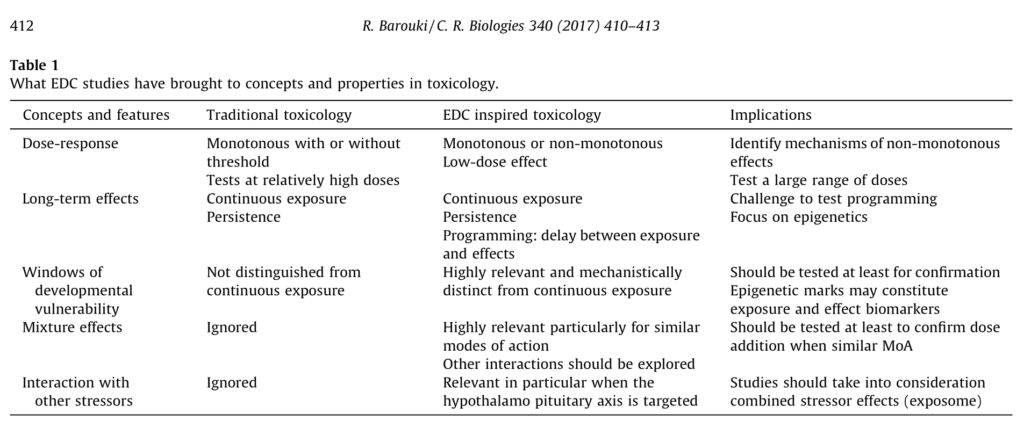
Legitimating the altered dose-response curve
Conventional toxicological approaches consider that as a given dose increases, the potential for a toxic, or harmful effect will increase as an upwards sloping dose response curve – as Paracelsus found in the 16th century (Grandjean, 2016).
Whereas nonlinear and even nonmonotonic relationships between dose and effect throw a spanner in the works. Hormones predominantly act at ppb and ppt rather than the parts per million (ppm) tested by regulatory authorities. However, for many EDCs, a larger dose at ppm may not be recognised by endocrine receptors. EDCs exploit this vulnerability, and are able to cause greater harm at a lower dose – following a non-monotonous dose response curve (NMDRC) – than at a higher dose. It’s important to recognise that NMDRCs may not be linear (see below).
The default position of presuming that toxicity only increases with the dose was challenged by Vandenberg and colleagues in their seminal paper Hormones and Endocrine-Disrupting Chemicals: Low-Dose Effects and Nonmonotonic Dose Responses (Vandenberg L. , et al., 2012). The paper comprehensively documented a wide range of studies which demonstrated greater effect from environmental chemicals at low, hormonally relevant levels, – levels much lower than that conventionally considered by regulatory communities.
Vandenberg and colleagues outlined the process of arriving at a safe level and the implications where regulators ignore nonmonotonic responses. When studies supplied to regulatory agencies arrive at a no observable effect level (NOAEL), it is assumed that no effect will occur below this. Regulators then undertake a series of calculations to derive a so-called safe dose, the reference dose. This may be 3 to a 1000 fold lower than the NOAEL, yet these levels are rarely tested. This process may be suitable for chemicals with standard dose response curves, but for those with nonmonotonic patterns, there is a risk conventional approaches can result in false negatives, i.e. concluding that exposure to the reference dose is safe when in fact it is not.
It’s important to remember that NMDRC is considered controversial in regulatory environments – but not in medical environments!
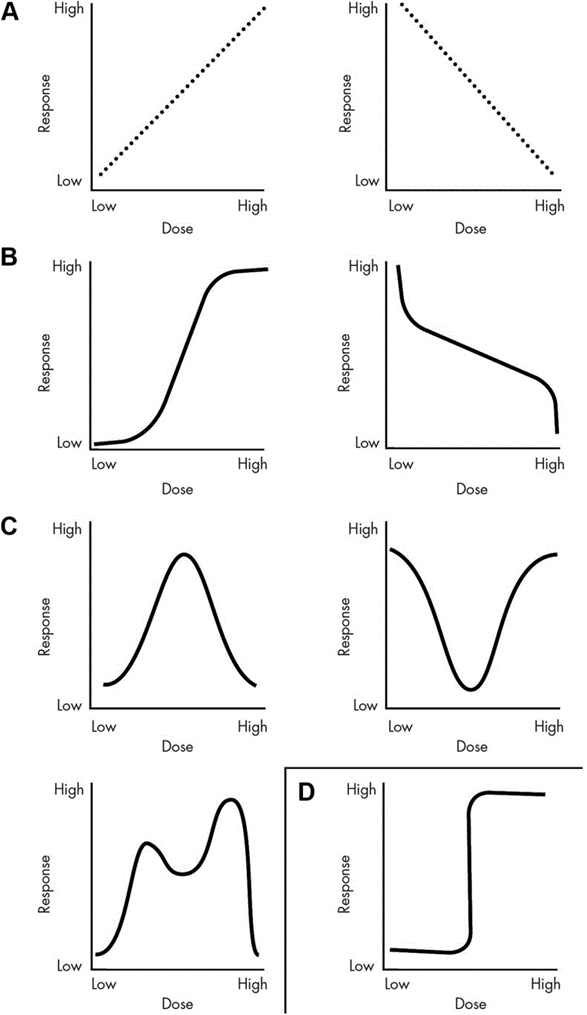
From (Vandenberg L. , et al., 2012): Examples of dose-response curves. A, Linear responses, whether there are positive or inverse associations between dose and effect, allow for extrapolations from one dose to another. Therefore, knowing the effects of a high dose permits accurate predictions of the effects at low doses. B, Examples of monotonic, nonlinear responses. In these examples, the slope of the curve never changes sign, but it does change in value. Thus, knowing what happens at very high or very low doses is not helpful to predict the effect of exposures at moderate doses. These types of responses often have a linear component within them, and predictions can be made within the linear range, as with other linear responses. C, Displayed are three different types of NMDRCs including an inverted U-shaped curve, a U-shaped curve, and a multiphasic curve. All of these are considered NMDRCs because the slope of the curve changes sign one or more times. It is clear from these curves that knowing the effect of a dose, or multiple doses, does not allow for assumptions to be made about the effects of other doses. D, A binary response is shown, where one range of doses has no effect, and then a threshold is met, and all higher doses have the same effect.
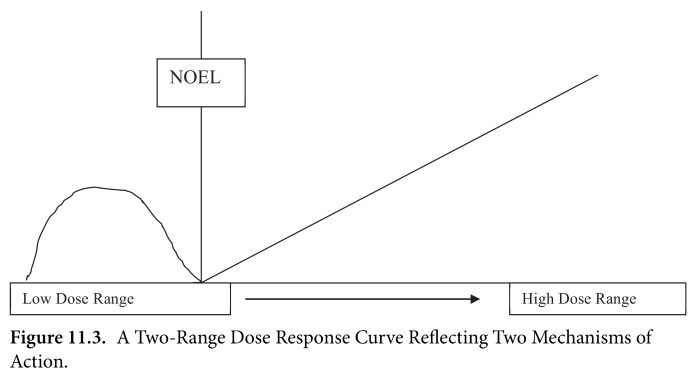
Krimsky’s simplified two-range dose response curve:
Establishing endocrinologic frameworks for regulation
Four types of studies have shaped the EDC field: studies (predominantly rodent) exploring the consequences of EDC exposures on development and physiology; mechanistic studies which unpick gene expression and epigenetic changes induced in cell and tissue culture, as well as research looking at molecular and cellular changes in endocrine tissues; research looking at body burdens and associated disease risks; and occupational and acute exposure reports concerning specific chemicals (Gore, et al., 2015).
As scientific data demonstrating mechanisms of action of EDCs has accumulated, scientists have established Key Characteristics (KCs) of EDCs:
‘[KCs] – common features of hormone regulation and action that are independent of the diversity of the effects of hormones during the life cycle’ (La Merrill, et al., 2019).
The identification of KCs are an important step in assisting the scientific community and chemical regulators to search for, identify and evaluate EDCs. To date ten KCs have been identified, and these can be updated as new knowledges concerning EDC activity arises. The authors of the Expert Consensus Statement emphasise that the current KCs are not a checklist, rather, universal framework for organizing mechanistic evidence for hazard identification that can be the foundation for the implementation of EDC risk assessments worldwide (La Merrill, et al., 2019).
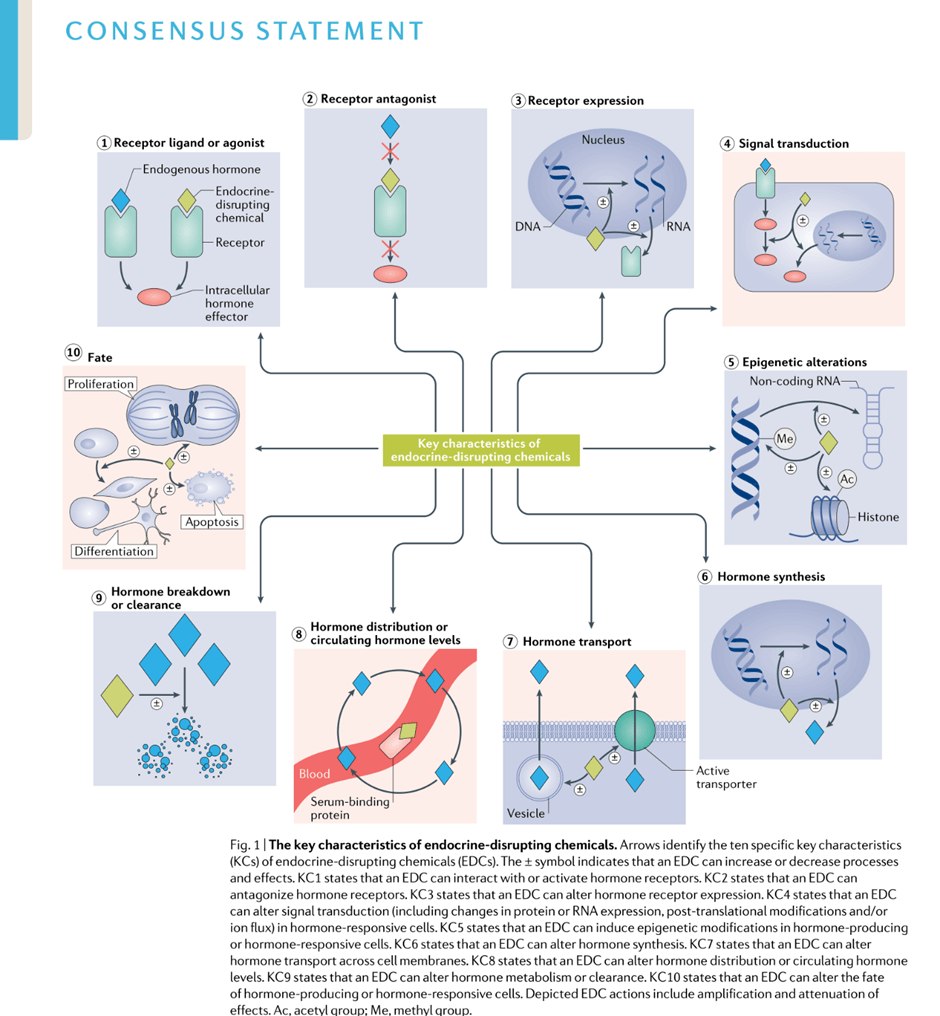
Scientific collaboration for a global independent effort
Kassotis and colleagues (2020) have highlighted the ad hoc, inconsistent and often out-of-date approach internationally, and suggested an integrative policy approach to improve risk assessment.
1. Integrate a two-tier screening system. Following Schug et al. (2013) establishment of a two-tier screening system to identify suspected and known EDCs. The first tier would utilise high-throughput screening methods to evaluate substances for a wide range of potential endocrinologic activity. In the second tier testing would utilise more sensitive assays to target critical endpoints and identify relevant critical windows.
2. Unblind the regulators. Recognise that if risk assessors use risk-based regulation (which, unlike hazard-based regulation does not remove dangerous chemicals from use once they are identified as harmful) exposures must be accurate. Therefore there is a greater obligation in risk-based jurisdictions to ensure robust biomonitoring data is supplied. Such data, accessible to researchers and organisations, can inform local and global institutions on exposure risk. In addition, if biomarkers and exposures are to be accurately characterised, industries should be required to disclose all the ingredients in retail formulations.
3. Improve oversight. Adopt a legally valid definition of EDCs, include economic costs of EDC-related health effects in GBD assessments; and adopt a hazard-based approach as a specific hazard category for EDCs when human exposures are confirmed. The hazard-based approach, once exposures are confirmed, is essential as it is in practice impossible to navigate the nuances of risk across human biology – particularly when age-related vulnerability is considered.
4. Establish an autonomous body, an International Agency for Research on EDCs (IARE) to produce collaborative reports describing ‘state of the evidence using three streams of evidence (eg, mechanistic, animal, and epidemiological studies) and principles similar to those used in systematic reviews. The IARE would be created within the WHO and modelled the International Agency for Research on Cancer (IARC) – or alternatively the IARC function would be broadened to integrate the evidence on EDCs – and funded similarly to protect against undue industry influence from industry. The IARE would not make policy recommendations. (Kassotis, et al., 2020).
With recognition of the slowness of progress, the environmental hypothesis approach has been separately proposed by scientists. The critical developmental period of pregnancy, infancy and children have been identified as a priority period for intervention. In this period, the need for protection and precaution is particularly critical due to the knock-on costs over the life course. The environmental hygiene hypothesis proposes policies and educational programs to promote a precautionary global protective approach. This is suggested because in absence of data on all exposures and particularly the potential for cumulative and synergistic effects from multiple classes of compounds to multiple organs to produce harm that potentially lasts for a lifetime, the most practicable step may be to simply take daily action to avoid environmental chemicals (Bourguignon, et al., 2019).
In Conclusion
With the increasing human and environmental costs of EDCs being documented – and in particular – that knowledge that harm in infancy and childhood can create profound disadvantages across the life course – the necessity of protective regulation that can actively prevent harm becomes more pressing. However, even when a substantial literature supports the withdrawal of a substance, regulators appear resistant to enacting laws which would withdraw a substance from the market (vom Saal & Vandenberg, 2020).
As natural hormones interact multifactorially across body processes – hormone disrupting chemicals can similarly affect multiple biological pathways in human bodies, and drive illness and bad health. This explains why endocrine disruption research is recognised as a multidisciplinary endeavour – it is highly creative, requiring novel cross-platform work to understand and untangle complex biological processes. It is not only important that interdisciplinary work is undertaken in the scientific community, it is important to create opportunities for EDC researchers to intersect with the medical community and with the broader political and business community (Schug, et al., 2016).
New Zealand’s absence of a quorum of scientists may explain the absence of EDC policy, however the reverse may also be true.
International scientist cohorts are working on this – but it is often extraordinarily difficult to secure funding. Many nation-states, including New Zealand, don’t prioritise this field of research. Unlike research that may promise a patent, and profit, for the investment firm, EDC research often directly challenges polluting organisations. Industry groups, may be closely allied with government departments, tied into policy development at local, regional and national level and have private public partnerships with academic institutions. Because it is multidisciplinary, the complexity can stymy funding bodies into inaction.
Often, scientists only enter this field by chance or by accident.
In the face of industry resistance and nation-state ambivalence, with recognition that science funding is strategically geared towards innovation and economic growth – not towards identifying the causes and consequences of economic growth – this research space is often filled by senior scientists with greater latitude to drive their own research, who exhibit curiousity, persistence and a tenacious values-based approach to uncovering environmental and human health harms in service of the public good.
Bibliography
Adegoke, E., Rahman, M., Park J-Y, Kim, YJ, & Pang, M.-G. (2021). Endocrine-Disrupting Chemicals and Infectious Diseases: From Endocrine Disruption to Immunosuppression. Int. J. Mol. Sci., 3939. doi:10.3390/ijms22083939
Alonso-Magdalena, P., & Nadal, A. (2020). The Commonly Overlooked Factor. Commentary on: “Environmental Obesogens and their Impact on Susceptibility to Obesity”. Endocrinology, 161(9), 1-2. doi:10.1210/endocr/bqaa123
Attina, T., Hauser, R., Sathyanarayana, S., Hunt, P., Bourguignon, J., Myers, J., . . . Trasande, L. (2016). Exposure to endocrine-disrupting chemicals in the USA: a population-based disease burden and cost analysis. Lancet Diabetes Endocrinol 2016; 4: 996–1003. Lancet Diabetes and Endocrinology, 4(12), 996-1003. Retrieved from https://www.thelancet.com/journals/landia/article/PIIS2213-8587(16)30275-3/fulltext
Barouki, R. (2017). Endocrine disruptors: Revisiting concepts and dogma in toxicology. Comptes Rendus Biologies, 340, 410-413. doi:10.1016/j.crvi.2017.07.005
Bourguignon, J., Parent, A., Kleinjans, J., Nawrot, T., Schoeters, G., & Van Larebeke, N. (2019). Rationale for Environmental Hygiene towards global protection of fetuses and young children from adverse lifestyle factors. Environmental Health, 17, 42. Retrieved from https://doi.org/10.1186/s12940-018-0385-y
Colborn, T., Myers, J., & Dumanoski, D. (1997). Our Stolen Future: Are We Threatening Our Fertility, Intelligence, and Survival? A Scientific Detective Story. Plume.
Comendant, C., & Davies, P. (2018). Economic assessment of paraquat use in New Zealand. Sapere Research Group. Retrieved from https://www.epa.govt.nz/assets/FileAPI/hsnoar/APP203301/c34071b227/APP203301-Appendix-B-Sapere-economic-and-benefits-assessment.pdf
Demeneix, B. (2017). Toxic Cocktail. How chemical pollution is poisoning our brains. New York: Oxford University Press.
Demeneix, B., & Slama, R. (2019). Endocrine Disruptors: from Scientific Evidence to Human Health Protection. requested by the European Parliament’s Committee on Petitions. PE 608.866 – March 2019. Brussels: Policy Department for Citizens’ Rights and Constitutional Affairs.
DeWitt, J., & Patisaul, H. (2018). Endocrine disruptors and the developing immune system. Current Opinion in Toxicology, 10, 31-36. doi:10.1016/j.cotox.2017.12.005
Gálvez-Ontiveros, Y., Páez, S., Monteagudo, C., & Rivas, A. (2020). Endocrine Disruptors in Food: Impact on Gut Microbiota and Metabolic Diseases. Nutrients, 12(1158). doi:10.3390/nu12041158
Ghassabian, A., & Trasande, L. (2018). Disruption in Thyroid Signaling Pathway: A Mechanism for the Effect of Endocrine-Disrupting Chemicals on Child Neurodevelopment. Front. Endocrinol. doi:10.3389/fendo.2018.00204
Gore, A., Chappell, V., Fenton, S., Flaws, J., Nadal, A., Prins, G., . . . Zoeller, R. (2015). 2015. EDC-2: The Endocrine Society’s Second Scientific Statement on Endocrine-Disrupting Chemicals. Endocr Rev, 36(6), E1-E150.
Grandjean, P. (2016). Paracelsus Revisited: The Dose Concept in a Complex World. Basic Clin Pharmacol Toxicol, 119(2), 126-132.
Grandjean, P., & Bellanger, M. (2017). Calculation of the disease burden associated with environmental chemical exposures: application of toxicological information in health economic estimation. Environmental Health123, 16.
Green, M., Harvey, A., Finger, B., & Tarulli, G. (2021). Endocrine disrupting chemicals: Impacts on human fertility and fecundity during the peri-conception period. Environ. Res., 194(110694). doi:10.1016/j.envres.2020.110694
Gupta, R., Kumar, P., Fahmi, N., Garg, B., Dutta, S., Sachar, S., . . . Vimaleswaran, K. (2020). Endocrine disruption and obesity A current review on environmental obesogens. Current Research in Green and Sustainable Chemistry, 3, 100009.
Hauser, R., Skakkebaek, N., Hass, U., Toppari, J., Juul, A., Andersson, A., . . . Trasande, L. (2015). Male reproductive disorders, diseases, and costs of exposure to endocrine-disrupting chemicals in the European union. J Clin Endocrinol Metab, 100, 1267-1277.
Heindel, J., Blumberg, B., Cave, M., Machtinger, R., Mantovani, A., Mendez, M., . . . vom Saal, F. (2017). Metabolism disrupting chemicals and metabolic disorders. Reprod Toxicol ., 68, 3-33. doi:10.1016/j.reprotox.2016.10.001.
Honkela, N., Toikka, A., Hukkinen, J., & Honkela, T. (2014). Coming to grips with scientific ignorance in the governance of endocrine disrupting chemicals and nanoparticles. Environmental Science and Policy, 154-163.
Hunt, P., Sathyanarayana, S., Fowler, P., & Trasande, L. (2016). Female Reproductive Disorders, Diseases, and Costs of Exposure to Endocrine Disrupting Chemicals in the European Union. J Clin Endocrinol Metab, 101(4), 1562-70. doi:10.1210/jc.2015-2873.
Iglesias-González, A., Hardy, E., & Appenzeller, B. (2020). Cumulative exposure to organic pollutants of French children assessed by hair analysis. Environment International, 134, 105332. doi:10.1016/j.envint.2019.105332
Iorns Magallanes, C. (2018). Permitting Poison: Pesticide Regulation in Aotearoa New Zealand. EPLJ, 456-490.
Kasonga, T., Coetzee, M., Kamika, I., Ngole-Jeme, V., & Momba, M. (2021). Endocrine-disruptive chemicals as contaminants of emerging concern in wastewater and surface water: A review. Journal of Environmental Management, 277, 111485. doi:10.1016/j.jenvman.2020.111485
Kassotis, C., Vandenberg, L., Demeneix, B., Porta, M., Slama, R., & Trasande, L. (2020). Endocrine-disrupting chemicals: economic, regulatory, and policy implications. The Lancet, 8, 719-730.
Kermath, B., Thompson, L., Jefferson, J., Ward, M., & Gore, A. (2022). Transgenerational Effects of Prenatal Endocrine Disruption on Reproductive and Sociosexual Behaviors in Sprague Dawley Male and Female Rats. Toxics, 10(47). doi:10.3390/toxics10020047
Kioumourtzoglou, M.-A., Coull, B., O’Reilly, E., Ascherio, A., & Weisskopf, M. (2018). Association of Exposure to Diethylstilbestrol During Pregnancy With Multigenerational Neurodevelopmental Deficits. JAMA Pediatrics, 172(7), 670-677. doi:10.1001/jamapediatrics.2018.0727
Krimsky, S. (2014). Low-Dose Toxicology Narratives from the Science-Transcience Interface. In J. Boudia, & N. Jas (Eds.), Powerless Science? : Science and Politics in a Toxic World (pp. 234-253). New York: Berghahn.
Kumar, M., Sarma, D., Shubham, S., Kumawat, M., Verma, V., Prakash, A., & Tiwari, R. (2020). Environmental Endocrine-Disrupting Chemical Exposure: Role in Non-Communicable Diseases. Front. Public Health. doi:https://doi.org/10.3389/fpubh.2020.553850
Kwiatkowski, C., Bolden, A., Liroff, R., Rochester, J., & Vandenbergh, J. (2016). Twenty-Five Years of Endocrine Disruption Science: Remembering Theo Colborn. Environmental Health Perspectives, A151-A154.
La Merrill, M. A., Vandenberg, L., Smith, M., Goodson, W., Browne, P., Patisaul, H., . . . Zoeller, R. T. (2019). Consensus on the key characteristics of endocrine- disrupting chemicals as a basis for hazard identification. Endocrinology, 45-57.
Metcalfe, C., Bayen, S., Desrosiers, M., Muñoz, G., Sauvé, S., & Yargeau, V. (2021). Methods for the analysis of endocrine disrupting chemicals in selected environmental matrixes. Environ. Res. doi:10.1016/j.envres.2021.112616
Nesan, D., & Karrasch, D. (2020). Gestational Exposure to Common Endocrine Disrupting Chemicals and Their Impact on Neurodevelopment and Behavior. Annual Reviews, 82, 177-202. doi:10.1146/annurev-physiol-021119-034555
NIH. (2010). President’s Cancer Panel. Reducing Environmental Cancer Risk. 2008–2009 Annual Report. National Institutes of Health and National Cancer Institute.
Nilsson, E., Sadler-Riggleman, I., & Skinner, M. (2018). Environmentally induced epigenetic transgenerational inheritance of disease . Environmental Epigenetics, 1-13.
NZEPA. (2018). Application Form: To obtain approval to import or manufacture a pesticide. APP203611 NUL3232 ‘Crucial’ herbicide. New Zealand Environmental Protection Authority. Retrieved from https://www.epa.govt.nz/assets/FileAPI/hsnoar/APP203611/c4686971d9/APP203611_Final_Application_Form.pdf
NZEPA. (2020). Risk Assessment Methodology for Hazardous Substances. New Zealand Environmental Protection Authority. Retrieved from https://www.epa.govt.nz/assets/Uploads/Documents/Hazardous-Substances/Risk-Assessment-methodology/Risk-Assessment-Methodology-for-Hazardous-Substances-How-to-assess-the-risk-cost-and-benefit-of-new-hazardous-substances-for-use-in-New-Zealand-v2.docx
NZEPA. (2021). Official Information Act Request. ENQ-40480-S3L2B9. Retrieved from https://www.epa.govt.nz/assets/RecordsAPI/OIA-response-22-January-2021-endocrine-disrupting-compounds.pdf
O’Shaunghnessy, K., Fischer, F., & Zenclussen, A. (2021). Perinatal exposure to endocrine disrupting chemicals and neurodevelopment: How articles of daily use influence the development of our children. Best Practice & Research Clinical Endocrinology & Metabolism, 35(5), 101568.
Palanza, P., Nagel, S., Parmigiani, S., & vom Saal, F. (2016). Perinatal exposure to endocrine disruptors: sex, timing and behavioral endpoints. Current Opinion in Behavioral Sciences, 7, 69-75.
Papalou, O., Kandaraki, E., Papadakis, G., & Diamanti-Kandarakis, E. (2019). Endocrine Disrupting Chemicals: An Occult Mediator of Metabolic Disease. Frontiers in Endocrinology, 10(112). doi:10.3389/fendo.2019.00112
Persson, L., Carney Almroth, B., Collins, C., Cornell, S., de Wit, C., Diamond, M., . . . Hauschild, M. (2021). Outside the Safe Operating Space of the Planetary Boundary for Planetary Boundaries. Environ. Sci. Technol. doi:https://doi.org/10.1021/acs.est.1c04158
Preau, L., Fini, J., Morvan-Dubois, G., & Demeneix, B. (2015). Thyroid hormone signaling during early neurogenesis and its significance as a vulnerable window for endocrine disruption. Biochim Biophys Acta, 1849(2), 112-121. doi:10.1016/j.bbagrm.2014.06.015
PSGRNZ. (2021). Submission: Inquiry into Supplementary Order Paper No. 38 on the Health (Fluoridation of Drinking Water) Amendment Bill. Physicians and Scientists for Global Responsibility Charitable Trust (PSGR). Retrieved from https://psgr.org.nz/component/jdownloads/send/1-root/73-21fdw
Rappaport, S. (2016). Genetic Factors Are Not the Major Causes of Chronic Diseases. PLoS One, e0154387.
Rappaport, S. (2018). Redefining environmental exposure for disease etiology. Systems Biology and Applications, 4(30).
Rappaport, S., & Smith, M. (2010). Environment and Disease Risks. Science , 460-461.
Rivollier, F., Krebs, M.-O., & Kebir, O. (2019). Perinatal Exposure to Environmental Endocrine Disruptors in the Emergence of Neurodevelopmental Psychiatric Diseases: A Systematic Review. Int. J. Environ. Res. Public Health, 16(1318). doi:10.3390/ijerph16081318
Schug, T., Abagyan, R., Blumberg, B., Collins, T., Crews, T., DeFur, P., . . . Myers, J. (2013). Designing Endocrine Disruption Out of the Next Generation of Chemicals. Green Chem., 15(1), 181-198. doi:10.1039/C2GC35055F
Schug, T., Johnson, A., Birnbaum, L., Colborn, T., Guillette, L., Crews, D., . . . Heindel, J. (2016). Minireview: Endocrine Disruptors: Past Lessons and Future Directions. Molecular Endocrinology, 1096.
Skinner, M. (2016). Epigenetic transgenerational inheritance. Endocrinology, 12.
‘t Mannetje, A., Coakley, J., & Douwes, J. (2018). Report on the Biological Monitoring of Sekected Chemicals of Concern. Results of the New Zealand biological monitoring programme, 2014-2016. Technical report number 2017-1. Centre for Public Health Research.
Tanner, E., Hallerbäck, M., Wikström, S., Lindh, C., Kiviranta, H., Gennings, C., & Bornehag, C.-G. (2020). Early prenatal exposure to suspected endocrine disruptor mixtures is associated with lower IQ at age seven. Environment International, 134, 105185. doi:10.1016/j.envint.2019.105185
Trasande, L., Zoeller, R., Kortenkamp, A., Grandjean, P., Myers, J., DiGangi, J., . . . Heindel, J. (2016). Burden of disease and costs of exposure to endocrine disrupting chemicals in the European Union: an updated analysis. Andrology, 565-572.
Vandenberg, L. (2019). Endocrine Disruptors and Other Environmental Influences on Hormone Action. In L. Welling, & T. Shackelford, The Oxford Handbook of Evolutionary Psychology and Behavioral Endocrinology (pp. 1-36). Oxford Handbooks Online. doi:10.1093/oxfordhb/9780190649739.013.
Vandenberg, L., Colborn, T., Hayes, T., Heindel, J., Jacobs, D., Lee, D.-H., . . . Myers, J. (2012). Hormones and Endocrine-Disrupting Chemicals: Low-Dose Effects and Nonmonotonic Dose Responses. Endocr Rev., 378-455. Retrieved from 10.1210/er.2011-1050
Vandenberg, L., Colborn, T., Hayes, TB, Heindel, J., Jacobs, D., . . . Zoeller, R. (2013). Regulatory Decisions on Endocrine Disrupting Chemicals Should be Based on the Principles of Endocrinology. .38:1-5. Reprod Toxicol(38), 1-5.
Vermeulen, R., Schymanski, E., Barabási, A., & Miller, G. (2020). The exposome and health: Where chemistry meets biology. Science, 392-396.
vom Saal, F. (1984). The intrauterine position phenomenon: effects on physiology, aggressive behavior and population dynamics in house mice. Prog Clin Biol Res, 169, 135-79.
vom Saal, F. (1989). Sexual differentiation in litter-bearing mammals: influence of sex of adjacent fetuses in utero. J Anim Sci, 67(7), 1824-40.
vom Saal, F., & Vandenberg, L. (2020). Update on the health effects of bisphenol A: Overwhelming evidence of harm. Endocrinology.
Watts, M. (2013). Poisoning our Future: Children and Pesticides. Auckland: Pesticide Action Network Asia and the Pacific. Retrieved from https://www.panna.org/resources/poisoning-our-future-children-and-pesticides
Waugh, D. (2019). Fluoride Exposure Induces Inhibition of Sodium/Iodide Symporter (NIS) Contributing to Impaired Iodine Absorption and Iodine Deficiency: Molecular Mechanisms of Inhibition and Implications for Public Health. Int. Jnl. of Environmental Research and and Public Health, 16(6), 1086.
WHO. (2013, May 24). Nutrition: Effects of iodine deficiency. Retrieved from World Health Organization: https://www.who.int/news-room/questions-and-answers/item/nutrition-effects-of-iodine-deficiency
Yang, P., Lin, B., Zhou, B., Cao, W., CHen, P., Deng, Y., . . . Zeng, Q. (2021). Sex-specific associations of prenatal exposure to bisphenol A and its alternatives with fetal growth parameters and gestational age. Environment International, 146(106305). doi:10.1016/j.envint.2020.106305